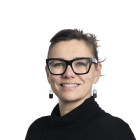
Energy FuturesPūngao mō Anamata
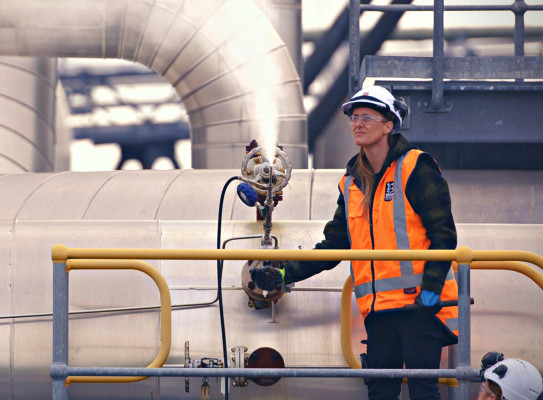
We deliver research and technology solutions that support a sustainable, low-carbon future for Aotearoa New Zealand.
Welcome to the future | Haere mai ki te Anamata
Imagine homes, businesses and transport powered by clean energy produced from above and below the ground, electric vehicles that automatically charge while they’re in motion and high-tech materials that cut costs while protecting the environment. Imagine putting our reliance on carbon-heavy fossil fuels (coal, oil and gas) firmly in the past and benefitting from a thriving planet filled with natural, renewable resources.
This is not just a dream of what’s possible in the future. It’s happening now.
Geothermal energy | Pūngao ngāwhā
Geothermal energy is renewable energy from the Earth’s core. It is abundant, sustainable, has a low environmental impact – and currently supplies around 20% of Aotearoa New Zealand’s electricity.
New Zealand’s geothermal fields are unique and ripe for further discovery. We will unlock more geothermal potential, both here in Aotearoa and in key locations around the globe.
Green hydrogen | Hauwai kākāriki
Hydrogen is the universe’s most abundant element, and green hydrogen is produced by splitting water into hydrogen and oxygen using renewably generated electricity. Hydrogen fuel cells convert hydrogen to clean energy as electricity for homes and businesses as well as powering cars, buses, trucks – and even rocket ships.
What is Green Hydrogen? – Green Hydrogen is an essential element for Aotearoa's carbon-neutral future transcript
Hydrogen is useful.
Green. For me, hydrogen is green.
Future, I'd say. It's going to be pretty important for climate change.
It's what we should be aiming towards. It's the tomorrow.
You could have your own storage of energy where you have solar panels then you store it in hydrogen and you can use that. Powering your TV, powering your kitchen.
Trains, trucks, planes, you're going to catch a flight, that will be powered by hydrogen, so you don't have to have that guilt of flying to a meeting and knowing that you're putting carbon emissions into the atmosphere.
Everyone has a hydrogen storage system so you can use hydrogen as an energy source rather than connecting to mains power.
It means that you are self sufficient, so you're not having to do all those things but pay the power bill!
All the fertilisers and steel we use, they will all be made without emitting carbon. Buses, ships, the planes we fly in. We will be doing the same things we usually do, but without emitting carbon.
We can produce hydrogen from water. We can then keep it for our own nation, or we can export it to another country.
Because we've got such a vast amount of green energy we can produce, New Zealand also has the potential to export that hydrogen overseas and convert New Zealand from a net energy importer to a net energy exporter. It could provide a lot of value to the entire country.
When you pass electrical energy through water you can actually split water into its constituents, hydrogen and oxygen. If that electricity comes from a renewable energy source that's called green hydrogen production.
You can think of hydrogen a lot like a little battery. Hydrogen stores energy within its bond. That energy can be released, for example through a fuel cell or hydrogen can also be combusted.
Engineers, physicists, chemists, social scientists you need diverse skills to think differently, to think outside the square.
We're trying to improve the efficiency of the hydrogen fuel cell.
How can you reduce the price? How can you reduce the energy that goes into building this?
We'll be using different kinds of water and seeing how that changes the process.
Potentially, it could work and do great solutions for the future. It might not - ultimately the science will determine what we do, but we need to explore that.
Technologies for a low-carbon future
We are changing molecular structures to create more durable and efficient materials that reduce energy demand for use in a wide range of applications. This new technology will support New Zealand’s transition to a low-carbon energy future.
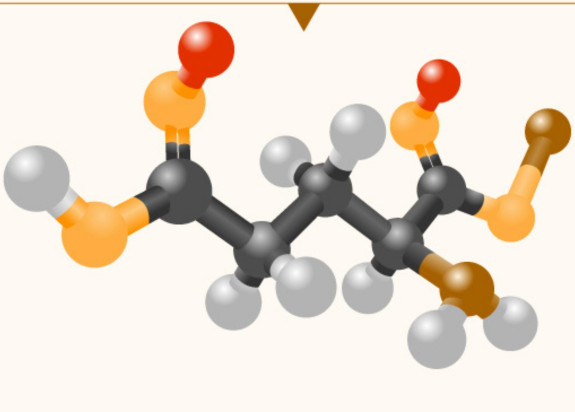
Our other research areas include:
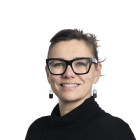
-
Our tohu (icon)
Our tohu (icon) – named Te Rā – has the sun, Tama-nui-te-rā, at its heart. The sun is a central figure of energy for most lifeforms here on earth, a symbol of hope, as with each new day comes potential and growth. Energy Futures looks forward, it is hopeful and carries the possibility for a better tomorrow. The two koru are the suns rays representing growth and expansion. This tohu speaks of hope, growth and potential.