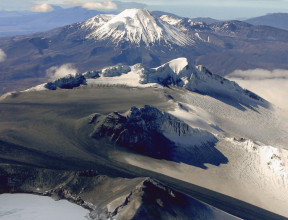
Volcanoes
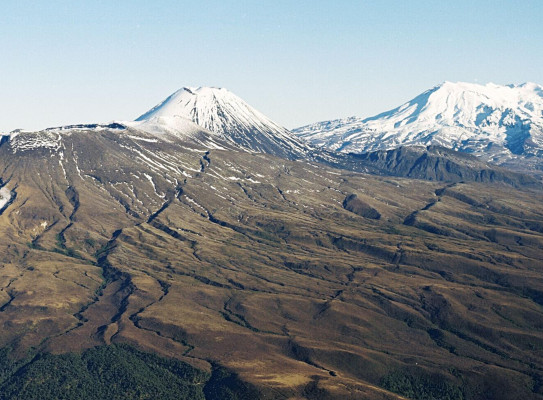
Our research on Aotearoa New Zealand’s volcanic landscapes provides useful information to responding agencies, infrastructure providers, fellow researchers and the public.
We also actively assist in incorporating our research into disaster management planning and building resilience in our communities.
Volcanoes are New Zealand’s landscape
Aotearoa New Zealand sits across the boundary between the Pacific and Australian tectonic plates, which is part of the ‘Pacific Ring of Fire’. This boundary is defined by the Hikurangi Trough off the east coast of the North Island and marks where the Pacific Plate descends under the Australian Plate. The volcanoes in New Zealand are fed by molten material (magma) produced by this process. Magmas are generated by a mix of partial melting of material from the Pacific Plate and the Australian Plate. These magma bodies accumulate and feed the active volcanoes above them.
Our volcanoes are world renowned, both in frequency of eruptions and volumes of material ejected. Familiar to the public, they dot the landscape of the North Island and extend in a line under the ocean along the Kermadec Ridge north-east towards Raoul Island and beyond. Today, many of these volcanoes are classed as active while some of the older ones are extinct. The time between eruptions can range from a few years to over a thousand years.
At GNS Science, we study extinct and active volcanoes to better understand what is happening in our dynamic landscape. Our research has applications in volcanology, preparedness, emergency response and community resilience.
Studying the past and present
Studying extinct and active volcanoes gives us insight into our volcanic past, present and future.
To look into the wonders of our volcanic environment our researchers:
- Use radioactive isotopes to date eruptions
- Link rocks to past eruptions to see how far material travelled
- Conduct geochemical analyses of material expelled to understand the state of the magma system driving the volcano when it erupted
- Examine the material from a particular site for clues to the duration, magnitude and length of each phase of an eruption
We also monitor present conditions of New Zealand’s active volcanoes by:
- Visual observations
- Chemical analyses
- Seismic and acoustic monitoring
- Ground deformation assessments
Data from monitoring of our volcanoes is fed into GeoNet. The volcano monitoring team gauge the present state of our active volcanoes and communicate this through the NZ Volcanic Alert Level system and through direct communication with stakeholders, responding agencies, infrastructure providers and the public.
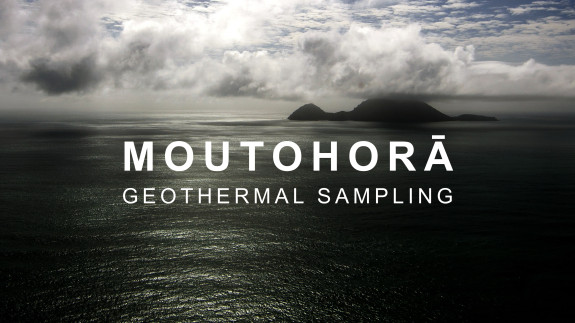
-
Applications for research
Studying volcanoes and past eruptions provide valuable insight into the timing, size and extent of past eruptions. This provides useful information for predicting and modelling future eruptions. These models inform our predictions of when an eruption may occur happen and how much damage it may cause.
Modelling is also used for creating hazard scenarios. If we can predict the timing, location, size and eruption style of a given volcano, we can predict hazards associated with it. These hazard scenarios are useful for emergencies services, lifeline groups and communities living near our active volcanoes for planning a response.
-
Mātauranga Maori
Māori oral history records may provide insight into eruptions of the recent past and into changes noted in the pre-eruption landscape. We work with Māori practitioners to provide a more holistic understanding of the behaviour of our maunga. Mātauranga Māori also informs the development of useful, culturally relevant disaster management plans and recovery plans that ensure mana whenua are supported through recovery and in developing resilience.